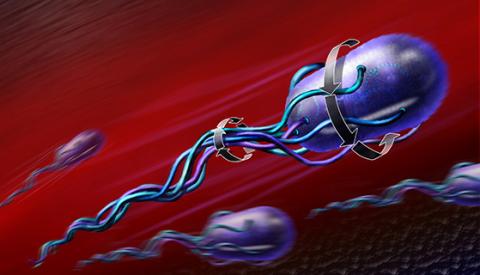
Artist’s representation of a bacteria with flagella (the long tendrils trailing behind), with arrows included denoting directional spin. (Nicolle Rager Fuller, via Microbe World, CC BY-NC-SA 2.0 DEED)
The lockdowns during 2020, the first year of the COVID-19 pandemic, temporarily paused most laboratory work, but they didn’t stop science from moving forward. Many scientists, including those comprising what’s now NCI’s Center for Structural Biology (CSB), seized the opportunity while out of the lab to revisit previously collected data.
Those efforts are paying off. The laboratory of CSB Chief Susan Lea, D.Phil., and collaborators has identified the precise mechanisms underpinning how bacteria change direction while moving through their environment. Having this understanding is foundational to biological and infectious disease research. The team’s study appears in Nature Microbiology.
They focused on a pair of protein complexes, known as the stator and the C-ring, that together form the biological motor attached to bacterial appendages called flagella. Flagella are what bacteria use to move: long, noodle-like structures that stick out and spin like a propeller.
Scientists have long known how flagella work. Past studies have shown that stators serve as miniature rotating cogs, turning the C-ring to spin a flagellum. A counterclockwise spin lets a bacterium “swim” forward, while a clockwise spin “tumbles” the bacterium, allowing it to stop its forward momentum and change direction. The activated form of a protein called CheY acts as a molecular gearshift by engaging and disengaging with the C-ring to switch between the two types of spinning.
But what scientists didn’t know is exactly how these motor molecules move as part of the process. It’s similar to understanding how a car’s engine functions but being unable to pinpoint certain motions that make it work.
This is where the team turned their attention. Their study reveals that the direction of the spin is dictated by the position of the stators relative to the C-ring. The counterclockwise spin occurs when the stators sit outside the C-ring. However, when the activated CheY engages, it changes the C-ring’s shape. This enables the stators to slip inside the C-ring, creating a clockwise spin.
“For many pathogens, such as Salmonella and E. coli, this movement is absolutely required for them to cause disease,” Lea explained via email, referring to bacteria’s flagella-driven propulsion.
Everything Old is New Again
The team’s insights come from a collection of old and new data. Part of the work spanned previous scans of flagella and their motor molecules via cryo-electron microscopy (cryo-EM), a powerful method for obtaining microscopic images at a near-atomic scale.
The team began revisiting some of the images while in lockdown, questioning whether they had more to offer. Meanwhile, as society reopened, they used their own cryo-electron microscope to take new images of the motor molecules from Salmonella bacteria. In time, they applied cutting-edge software and algorithms to the old images. These calculations helped further clarify the motor molecules’ structure and movements.
“We were able to combine our high-resolution C-ring structures with the even higher-resolution structure of a stator fused to a C-ring component, thanks to overlaps between them. Then we compared this composite with our earlier flagellar structures and placed them into the low-resolution images that other groups have taken within the cell,” said Steven Johnson, D.Phil., a staff scientist in CSB and co-first author on the study. “You can build up a high-resolution picture by combining all of these different approaches.”
Looking at a complete structure was important, said Justin Deme, Ph.D., a staff scientist in CSB and co-first author on the study. The answers gained by the team’s approach wouldn’t have been possible with a less comprehensive method.
“We’re moving towards a more natural approach to understand how the system works by imaging the components as a larger assembly. We’re not targeting specific proteins within this complex. It’s the interplay of these components that tells us how the system works,” he said.
Doing the work was far from simple. On top of the usual precision that cryo-EM requires, the team also had to deal with the molecules’ instability during imaging. The proteins are meant to move and change shape, so to ensure they’d hold together for imaging, the team developed multiple in-house methods to keep them in stable, relevant configurations for microscopy.
There was also the challenge of working amid a pandemic—and a transatlantic move. In 2020, Lea’s laboratory relocated from the University of Oxford, where the study began, to NCI Frederick. That’d be difficult under normal conditions, but the pandemic-era travel restrictions and supply chain backlogs added considerable complications.
“The flipside of that was some of the laboratory downtime did give us a bit of chance to reassess some of this old data,” Johnson said. “So, it was a challenge, but we got good things out of it.”
Deme agreed, adding that the pause on active laboratory research gave them a chance to assess projects’ directions and consider where to go next.
“There's not much you can do about a pandemic, but we tried to make the most of it,” he said.
Samuel Lopez leads the editorial team in Scientific Publications, Graphics & Media (SPGM). He writes for newsletters; informally serves as an institutional historian; and edits scientific manuscripts, corporate documents, and sundry other written media. SPGM is the creative services department and hub for editing, illustration, graphic design, formatting, multimedia, and training in these areas.