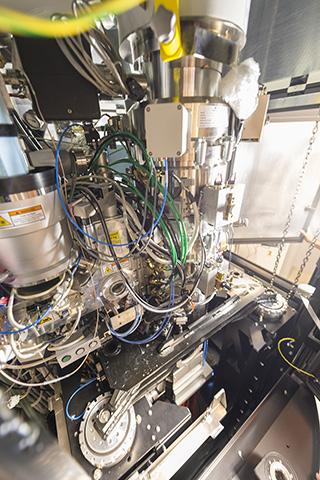
Inside a Titan Krios.
Editor’s note: This article is part of a series about scientific devices in use at NCI at Frederick and the Frederick National Laboratory. Other parts of the series can be found here. Mention of trade names, commercial products, or organizations does not imply endorsement by the U.S. government.
Every Monday morning, Ulrich Baxa, Ph.D., and his colleagues enter their Gaithersburg, Md., laboratory and begin calibrating their Titan Krios, a massive, $7-million transmission electron microscope that can capture high-definition images at near-atomic magnification. They load several flash-frozen biological samples into the Krios and, by 5 p.m., program the instrument to collect data. As the team leaves for the evening, the Krios begins shooting beams of energy into the samples and taking photos.
The Krios in Gaithersburg is one of three transmission electron microscopes in the NCI National Cryo-Electron Microscopy Facility (NCEF), which Baxa and his team operate as a national resource for cancer researchers. The other two, a Glacios and a second Krios, were installed this summer at the Frederick National Laboratory but are not fully operational.
Baxa and his team use the Gaithersburg Krios to perform cryo-electron microscopy (cryo-EM) on samples submitted by scientists at external institutions. Cryo-EM is a method for examining molecules and objects at a high resolution, capturing 2D images, and assembling a 3D structure of the molecules by back-projecting thousands of the 2D images on a computer.
Cryo-EM works for many types of macroscopic and microscopic objects, ranging from cancer-causing proteins to root canals and nematodes. It has proven so groundbreaking that three scientists won the 2017 Nobel Prize in Chemistry for their work to develop the method over several decades.
“Together these [microscopes] enable high-resolution structures of cancer targets,” said Baxa, NCEF’s senior microscopist. “Once you get a high-resolution structure, it’s much, much easier to use it as a drug target.”
The Krios microscopy process varies depending on the sample, but there are common elements. Before going into the microscope, samples are suspended in a liquid buffer that gets slathered onto a copper mesh coated with a pockmarked carbon film. Scientists plunge the mesh into liquid ethane, freezing the sample so quickly that the buffer doesn’t form ice crystals as it hardens. It instead becomes clear and glassy, which prevents the samples from becoming distorted and makes them appear as though they were still suspended in liquid.
Several grids are then loaded into a copper cartridge and inserted into the Krios, which in turn maintains them at approximately negative 310 degrees Fahrenheit. A robotic arm inside the Krios selects a grid, moves it into the microscope column, and places it onto the stage that sits above the Krios’ camera and scanner. The Krios zooms in on a miniscule section of the buffer that has frozen overtop one of the pockmarks in the film, then bombards it with electrons. Meanwhile, the camera takes a photo, capturing a high-quality 2D image due to the electrons passing through the sample.
Cryo-EM has been particularly useful in examining hard-to-image biological molecules, like proteins on the surface of cells. Unlike X-ray crystallography and other imaging techniques, it doesn’t rely on crystals or other methods that could unintentionally change the molecules’ structure, and the freezing process doesn’t risk altering the samples’ appearance under the microscope. This preserved accuracy is crucial for drug development studies that require 3D structures of samples as they appear in nature.
According to Baxa, the Krios is a top-of-the-line instrument for performing such work because many of its features were designed with cryo-EM in mind. It comes standard with the ability to attach a direct electron detector, which is extremely important for collecting such high-resolution data. The stability of the mechanical microscope stage and the lens design also help.
Because the Krios is so complex, every data collection session at the NCEF takes approximately eight hours to set up, program and align the microscope, and test the sample quality. Data collection lasts two to three days and results in 1,200–4,000 captured images.
The recently installed Glacios functions similarly to the Krios and shares many of its features, such as the ability to attach a direct electron detector. However, it is smaller, less powerful, and cheaper, making it ideal for optimization, sample screening, and studies that don’t require Krios-quality high-definition images.
The Glacios will allow the NCEF to prescreen samples before they run on the Krios, which should free up more time on the Krios for data collection. Adding to the convenience, the Glacios and the Krios use the same cartridge, so samples can be transferred between them without additional preparation.
The NCEF also plans to test an off-label method on the Glacios, called “micro-electron diffraction.” They will load the grids with microscopic crystals that contain biological and chemical molecules. By hitting them with the Glacios’ electron beam and taking pictures, they may be able to observe the molecules’ 3D structures. There’s no guarantee that it will work, but if it does, it will be faster, cheaper, and more convenient than conventional methods like nuclear magnetic resonance imaging or X-ray crystallography.
“If [you] can do that in a matter of hours … even if it’s less than a day, … that’s amazing,” said Baxa. “It could basically replace [nuclear magnetic resonance] for chemists.”
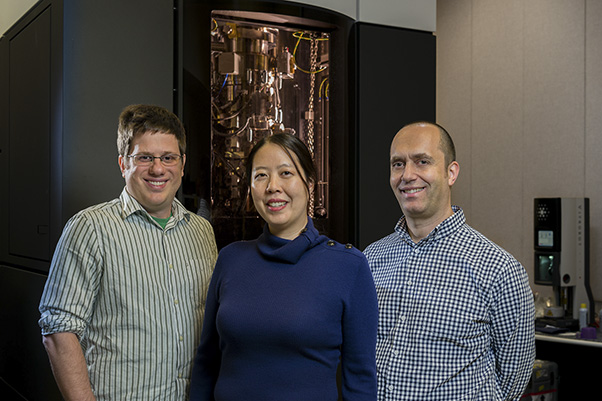